General Background
Optical nanospectroscopy uses ultraviolet (UV), visible, and near-infrared (NIR) light sources for obtaining nanoscale information via the interaction of light and matter. It offers unique possibilities of probing nature at the nanoscale with extremely high spatial, temporal, spectral, and/or chemical resolution. Nanospectroscopy encompasses the application of spectroscopic techniques with nanoscale resolution, techniques for the detection and spectral analysis of nano-objects or nano-patterns, and processes that take place at the nanoscale. It can be applied to metallic, semiconducting, or molecular systems that can be prepared using bottom-up or top-down strategies, and even living cells. Nanospectroscopy methods have seen tremendous improvement over the last two decades, boosting their sensitivity down to the single molecule level. Such methods have since been applied e.g. to visualize the distribution of chemical components or strain, to monitor energy and charge flow in composite materials or between single nano-objects, and to access biological processes on a sub-cellular level. These novel insights can be exploited, amongst others, for improving photovoltaics and energy efficiencies, engineering materials with desirable surface properties, or evolving targeted drug delivery. State-of-the-art UV/Vis/NIR nanospectroscopy is thus an invaluable tool that allows us to address a wide range of crucial issues in biotechnology, medicine, materials engineering, energy, or light harvesting. These big issues however cannot be solved by a single research group alone.
Optical nanospectroscopy is a method-based approach. In order to make substantial scientific progress by using nanospectroscopic techniques, a joint effort by all parties concerned is required. This includes experts in the preparation of novel sample materials (nanoparticles, quantum dots, bio samples, nanostructures, molecular engineering, hybrid structures, opto-electronic devices), experts in instrumentation (including relevant industry), experts in performing the elaborate nanospectroscopic experiments (Raman or near-field spectroscopy, pump-probe / time-resolved experiments, dark field / absorption spectroscopy, ...), and experts in data analysis and modelling. Strong expertise on these different aspects of nanospectroscopy exists all across Europe. The COST Action NanoSpectroscopy is intended to bring together this expertise in a broad interdisciplinary, multinational European effort.
This NanoSpectroscopy Action results from a longstanding collaboration between groups from several COST countries. These groups have previously organized joint schools, workshops, and bilateral visits. However, financing these events, and especially the participation of Early Stage Researchers (ESRs), has always proved difficult. This core group therefore now aims to strengthen their networking activities and increase the number of discussion partners. Here the big advantage of carrying this project out within the COST framework, rather than in the more individual research project centred alternative European programs, becomes apparent. The COST Action will establish contacts and links between researchers from the above fields, collect them in one joint Action, and attract additional contributors which the core group may not have been aware of before. With the help of the COST funding for networking and outreach activities, the Participants can meet and discuss new ideas, mutually visit laboratories, synergistically share equipment, exchange students, learn about new techniques hands-on, discuss with industry, exchange samples and identify future research projects. Without the COST Action, networking would always be subject to the initiative of individual researchers, restricted to a much smaller target group, and entirely depending on the availability of individual national or third-party travel funding.
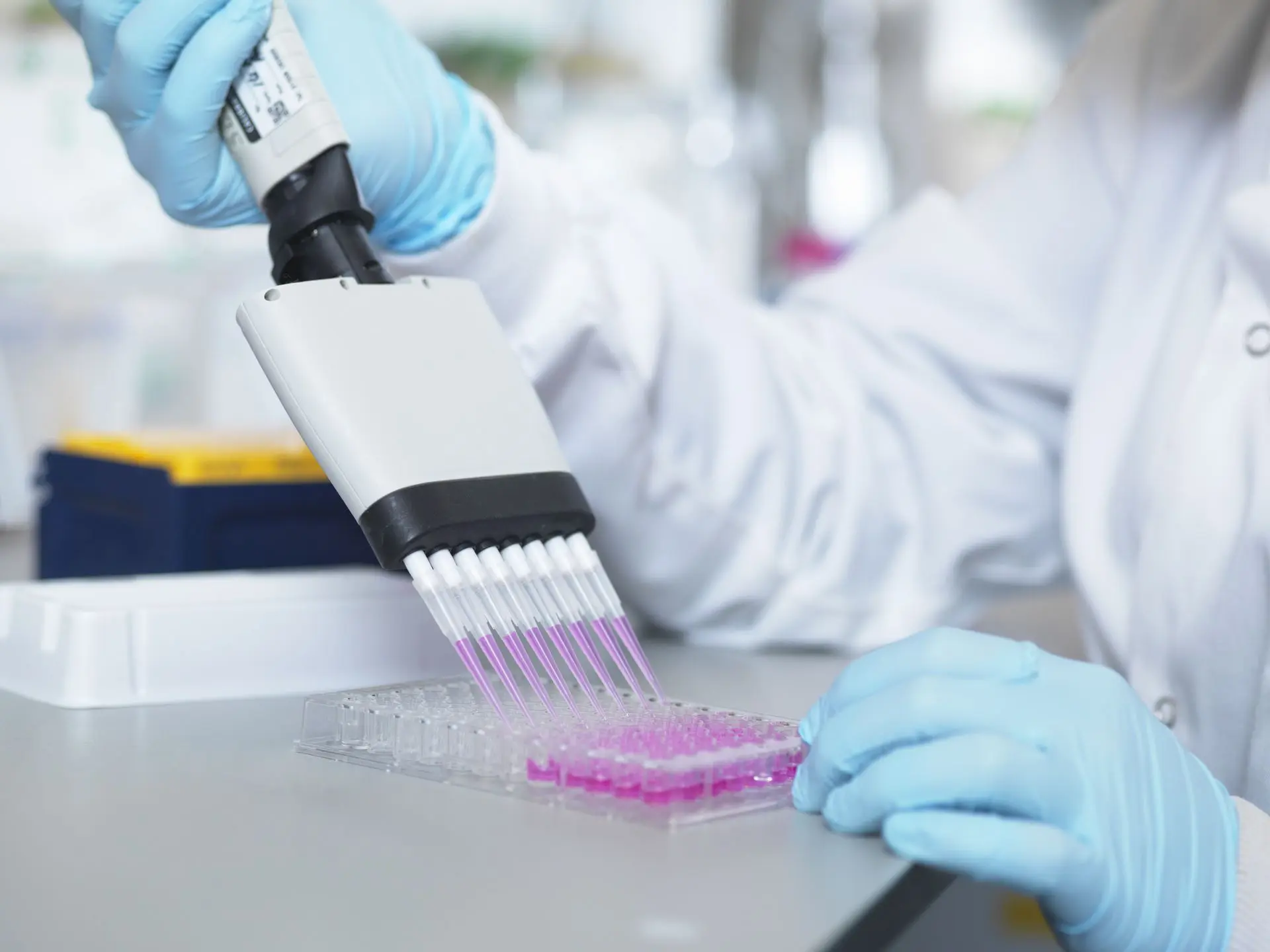
Current State of Knowledge
Two major directions of nanospectroscopy research are geared towards developing high-resolution measurement techniques, and towards applying these techniques for gaining physical, chemical or biological information at the nanoscale. Over the last few decades, optical spectroscopy has managed to reach sub-wavelength spatial resolution in combination with sub-wavenumber spectral accuracy and simultaneous topographic mapping. Time-resolution has been pushed towards femtosecond pulses, offering time-resolved spectra with high signal-to-noise ratio. Currently, confocal and time-resolved fluorescence, transient absorption, and surface- and tip-enhanced Raman spectroscopy, as well as dark-field scattering and absorption spectroscopy are applied to probe the spectral features of objects with high spatial, spectral, and/or temporal resolution. Nanospectroscopy allows for monitoring material properties, chemical reactions, or physical effects down to the single molecule level and a spatial resolution below 10 nm. The main systems that nanospectroscopy is currently applied to are optical nanoantennas, quantum dots / nanocrystals, single molecules / fluorophores, surfaces of material blends, and biological systems / cells.
Current research programs within the EU framework are aimed at employing nanospectroscopy for high-sensitivity biosensing, ultra-high resolution imaging for medicine and biology, applying theses techniques to new areas of application like lanthanides and actinides (the f-elements) or geological samples, at improving large-scale application, reproducibility, and data analysis routines, devising calibration standards for Raman spectroscopy, and at improving light sources and filters. Given the considerable equipment requirements of nanospectroscopy, research is mostly confined to Europe, the USA, and parts of Asia. European and US research are competing e.g. in the fields of nanospectroscopic sensing and high-resolution techniques, with many strong groups located in Europe. Many leading nanospectroscopic equipment vendors with strong R&D activities are based within Europe, but have strong competition in their US counterparts. Thus, the synergistic efforts within the COST Action will push European competitiveness in a future key technology.
The present Action will expand the range of systems that are investigated by nanospectroscopic means, using nanofabrication and molecular engineering approaches to devise novel hybrid composites and devices. It will bring innovation by evolving existing techniques to further improve their signal intensity, contrast, sensitivity, and robustness. State-of-the-art optical nanospectroscopy will specifically be addressed to the global problems of energy efficiency, light harvesting, and health care.
Reasons for the Action
The prime reason for the COST Action NanoSpectroscopy is the conviction that by promoting a Europe-wide dialogue and network between key actors in nanospectroscopy across different disciplines, countries, techniques, and targeted applications, as well as between academia and stakeholders in industry, the powerful techniques which form the basis of nanospectroscopy can be much more efficiently employed, more broadly distributed, and more key questions of scientific, industrial, and societal interest can be identified for which nanospectroscopy can find an answer. Optical spectroscopy at the nanoscale emerged in the late 1980s to early 1990s, and much progress has been made to date in understanding aspects of the behaviour of nano-materials. Still, many technical limitations and open questions remain, such as: How can sensitivity be further improved and single nano-objects be selectively addressed? What are the processes involved and the emerging properties when different types of nano-systems are coupled? What added value can hybrid nano-systems bring for technological applications? Current scientific endeavours tend to lead to segregation into separate communities according to the types of nanostructure under investigation. This COST Action will aim to promote a cohesive community across Europe that seeks to establish/maintain a world-leading position for European nanospectroscopy.
In many respects, nanospectroscopy techniques are still restricted to research laboratories, since they require elaborate equipment, expert knowledge on data analysis, and are not yet optimized for large areas, extreme conditions, or minimum time consumption. To make these promising techniques more accessible, a network of experts from fundamental and applied research as well as R&D is required. The COST Action scheme was therefore identified as an ideal means to promote European dialogue. Significant potential is perceived for technology transfer and collaboration with industry, in which Europe can lead the way if sufficient resources are allocated for co-ordinating the research efforts.
The objectives of this Action are to advance nanospectroscopic techniques, knowledge on the nanoscale properties of novel materials, and the distribution of nanospectroscopy. The pursuit of these objectives is expected to result in instrumentation and approaches with improved performances that are easier to operate for a broader set of users, and in the synthesis and fabrication of novel nanomaterials. The means needed to achieve them include the exchange of knowledge and ideas on different methods and analyte systems, from which new fruitful combinations and collaborations will arise. It is a crucial goal of this COST Action to engage in a dialogue with relevant industry partners. The Action is convinced that the improvement of existing nanospectroscopy techniques and equipment, in terms of stability, ease of data analysis and broader applications, will promote the implementation of these techniques by industry.
As future benefits, a new generation of nanospectroscopy researchers will be trained within the Action. New basic knowledge on the fundamental processes of life, material properties, and energy transfer processes will be gained, which will be of great significance for future applications. Key challenges currently faced by European societies can thus be addressed in the fields of energy efficiency (solar cells, batteries, LEDs, sensors, material design), healthcare/medicine for an ageing society (drug design and targeted delivery, early disease detection), or material design (stability, hydrophobicity, low friction, high intensity yield, cost effectiveness, ...). The short-term goals are mainly aimed at scientific and technological advances, while the long-term economic and societal benefits of the results may prove to be considerable as well.?
Aim
The COST Action NanoSpectroscopy aims at gathering European expertise in order to further develop UV/Vis/NIR/Raman nanospectroscopic techniques and modelling with (ultra-)high spatial, temporal, and spectral resolution and sensitivity, and the application of these techniques to novel (hybrid) (in)organic nanomaterials, complex device structures, and biosystems. The synergistic efforts in the interdisciplinary environment of the Action promise rapid progress in designing new nanospectroscopic techniques, further technical development of established techniques (e.g. towards non-specialist user-friendly setups), theoretical understanding and data processing, basic knowledge of light-matter interaction and energy flow at the nanoscale, and a boost in the manipulation of complex nanosystems, to be made available to societal needs.
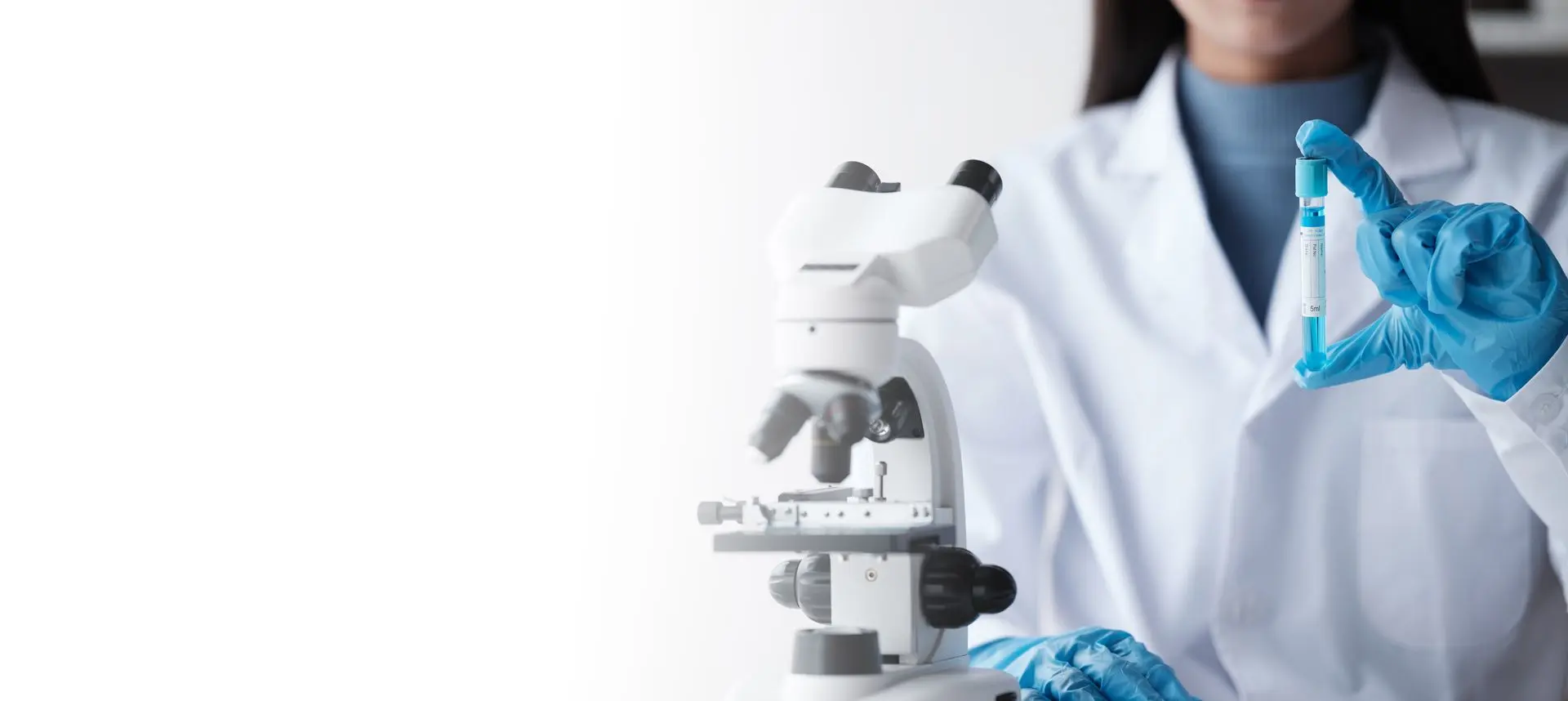
Objectives
As the main objective of COST NanoSpectroscopy, a multi-site, multidisciplinary European training and collaboration network will be established, which will cohesively combine aspects generally addressed as separate topics in the field of nanospectroscopy: improving spectroscopic techniques and instrumentation towards (ultra-)high resolution and sensitivity as well as user-friendly (turn-key) techniques, engineering molecular structures or nanoparticles, applying them for gaining basic knowledge on light-matter-interaction and for improving optical applications/devices, and theoretical modelling. The main objective is to make nanospectroscopy available to a broader range of topics, materials, and end users. As scientific objectives, organic layers and molecules with novel properties will be created by molecular engineering, and novel hybrid nanosystems (organic-inorganic, semiconducting-metallic, metallic-metallic) will be fabricated using nanotechnology. These nanosystems will be spectroscopically investigated. A particular focus will be on analyzing energy and charge flow and light harvesting at the nanoscale, to improve materials for applications e.g. in photovoltaics or sensing/medical diagnostics.
Outreach activities will make the new developments accessible to a wider audience. The COST Action will serve as a nucleus for developing joint proposals, from bilateral collaborations to European projects.
Early Stage Researchers (ESRs) will be trained in joint research projects, Short-Term Scientific Missions (STSMs), hands-on training, and training schools organized by ESRs for ESRs. Stronger links between industry and academia are expected to trigger joint instrumentation development. A list of current open issues in nanospectroscopic instrumentation and data analysis development will be drafted in communication with industry and disseminated via the Action's public website. Each year, one joint COST NanoSpectroscopy workshop will be organized. The objectives of these activities are synergies from interdisciplinary work between Experts from different areas. Well-trained ESRs will be able to identify the next generation of scientific topics. A coherent textbook on nanospectroscopy will be written and published by the Participants. The COST framework is ideally suited to reach the above objectives, since it enables reducing fragmentation in the field of nanospectroscopy and connecting groups using different techniques with groups working on different model systems in a concerted Action. It constitutes a logical extension of the applicants' existing informal network to the European level.
How Will Networking within the Action Yield the Objectives?
Networking within the Action will bring together the necessary expertise from the different areas of the diverse communities that are working with/on nanospectroscopy. Interdisciplinary collaborations cross-linking materials design with nanospectroscopic techniques will lead to fresh approaches and open up additional fields of application for nanospectroscopy, and enlarge the end user community. By promoting communication, bi- and multilateral research projects and technology transfer will emerge. By integrating ESRs into the networking activities, a new generation of nanospectroscopy experts will be trained.
Potential Impact of the Action
One direct outcome of the project will be a coherent textbook on optical nanospectroscopy. The research results and networking activities will be made accessible via a public website. This Action will advance current knowledge on processes such as energy flow and light-matter-interaction at the nanoscale and lead to innovations in interdisciplinary areas across (bio-)physics, chemistry, biology, nano-/materials science, and engineering. Knowledge about the preparation and properties of novel samples, in particular hybrid combinations of components, will be gained. Novel specifically designed supramolecular structures and nanostructuring techniques will result from the Action and have a wider impact. The limits of the spatial and temporal resolution that can be achieved with nanospectroscopy will be pushed further. Potentially new levels will be reached in the advancement of devices such as photovoltaic cells, LEDs, biosensors, or spasers. Nanospectroscopy techniques will be further developed with respect to performance and user-friendliness. By addressing technology-transfer issues, COST NanoSpectroscopy is expected to impact the dissemination of nanospectroscopy techniques beyond the research laboratory and make them more accessible to general users. As a facilitator for advances in fields like energy efficiency, materials science, light harvesting, optoelectronics, and medicine, its long-term societal impact promises to be considerable.
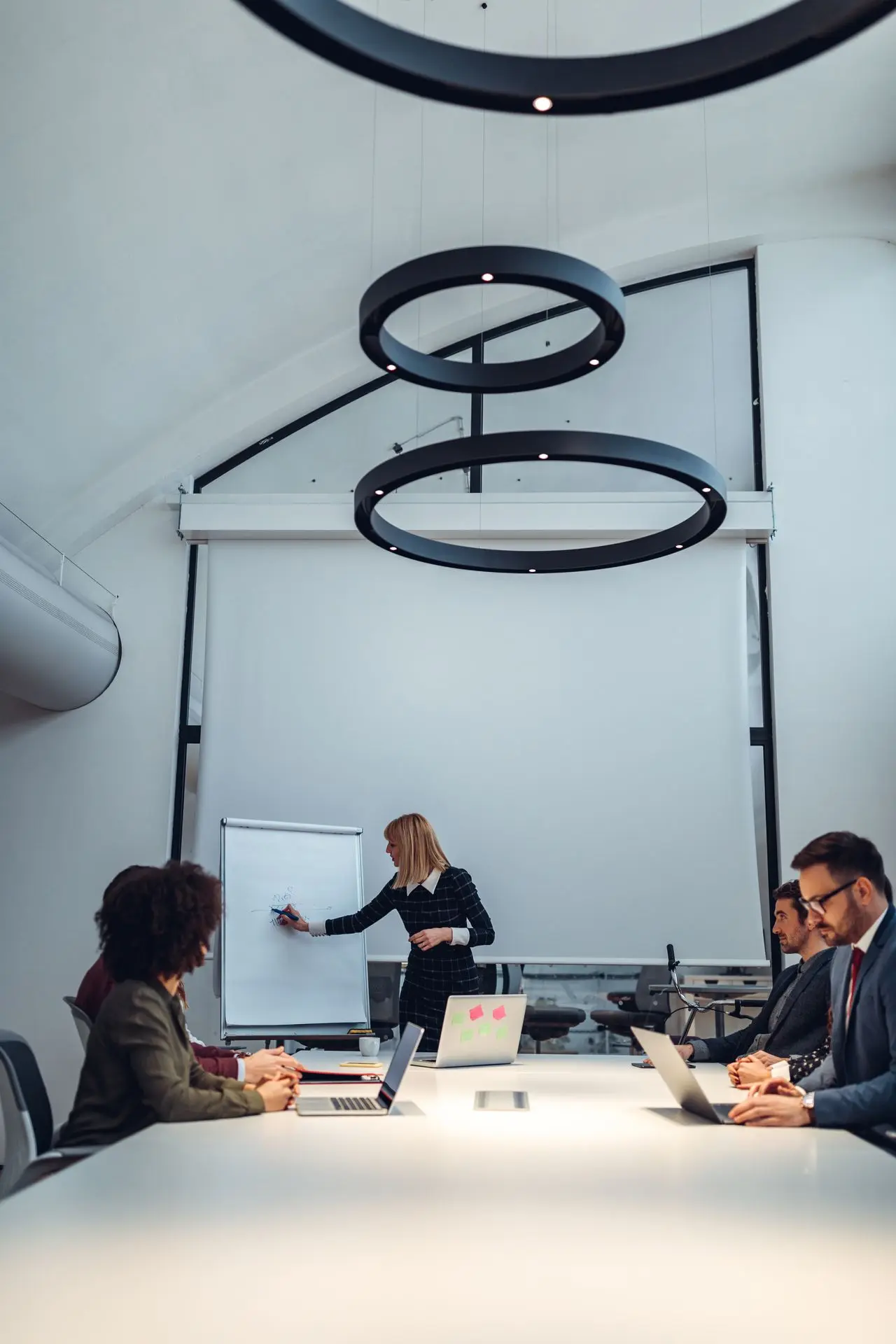
Target Groups and End Users
Academia: Knowledge on technical issues, basic science, energy aspects, material properties, and physico- chemical effects at the nanoscale will be increased. By dissemination and training, a new generation of academics is instructed. Both established and junior researchers were involved in preparing the Action.
Industry: Connections between academia and industry will be strengthened for instrument development, training, and wider applications (e.g. development of turn-key nanospectroscopy techniques), with the possible creation of spin-off companies. An R&D institute was involved in preparing this Action.
Interested general public: The knowledge obtained in the context of this COST Action will be disseminated through a dedicated website, talks at international conferences, and a coherent textbook on nanospectroscopy.
Long-term societal benefit: This Action will pave the way towards improvements in areas of strong societal impact, such as energy efficiency, materials and medical development, or light-to-energy-conversion, and increase general knowledge on topics of broad interest like the basic processes of life.
Coordination and Organisation
The COST Action NanoSpectroscopy is chaired by an Action Chair and a Vice Action Chair. It is represented by the Management Committee according to the Rules and Procedures for Implementing COST Actions. Each participating institution should name one Representative to the Action Chairs as their contact person. The Action will be actively advertised. Apart from Management, four Working Groups will be set up to address the areas "System design and nanofabrication", "Physical processes and modelling", "Improvement of spectroscopic techniques" and "Preparation of a textbook".
The individual research activities and projects within COST NanoSpectroscopy will be initialized, defined, funded and organized by the participating scientific groups/countries. COST NanoSpectroscopy will promote and coordinate the networking and dissemination activities and communication between the Participants. Research links and new project ideas across the different techniques, materials, applications, and countries will be established within the WGs. For each of the WGs, one WG Leader and one Vice WG Leader will be elected by the MC. Each Participant should indicate his/her participation in one or more WGs.
As milestones of COST NanoSpectroscopy, annual workshops will be organized where new scientific results and directions will be discussed. A database of European nanospectroscopy activities will be established, an Action list of current issues in nanospectroscopy instrumentation development will be compiled, and a coherent textbook on nanospectroscopy will be published. In terms of materials, the Action expects to develop improved techniques for the fabrication of nanostructures and position-controlled integration of individual optically-active species (quantum dots, molecules, core-shell nanocrystals, di- and oligomers, etc.). The efforts in theoretical modelling aim to establish new toolboxes for the understanding of energy flow at the nanoscale, usable not only by theorists but also by experimentalists. The targeted refinement of nanospectroscopic techniques and near-field probes, honed by investigations of the aforementioned novel nanomaterials, can be expected to make spectroscopic techniques with ultra-high spatio-temporal resolution available to a greater number of partners in academia and industry. A dedicated website will be set up for the COST Action NanoSpectroscopy. The contents will be updated at least once every three months to include a current list of the Participants, references to new publications by the Participants, short articles, announcements of upcoming COST activities, and links to information for the general public.
The annual NanoSpectroscopy workshop will be scheduled to take place in a different country each year. For this purpose, national teams will be appointed to be in charge of local organization. For practical purposes, some single-day hands-on training courses and industry dialogue meetings may be mostly targeted but not restricted to researchers within a certain travelling radius. Reports of these activities will however be posted on the COST NanoSpectroscopy website.
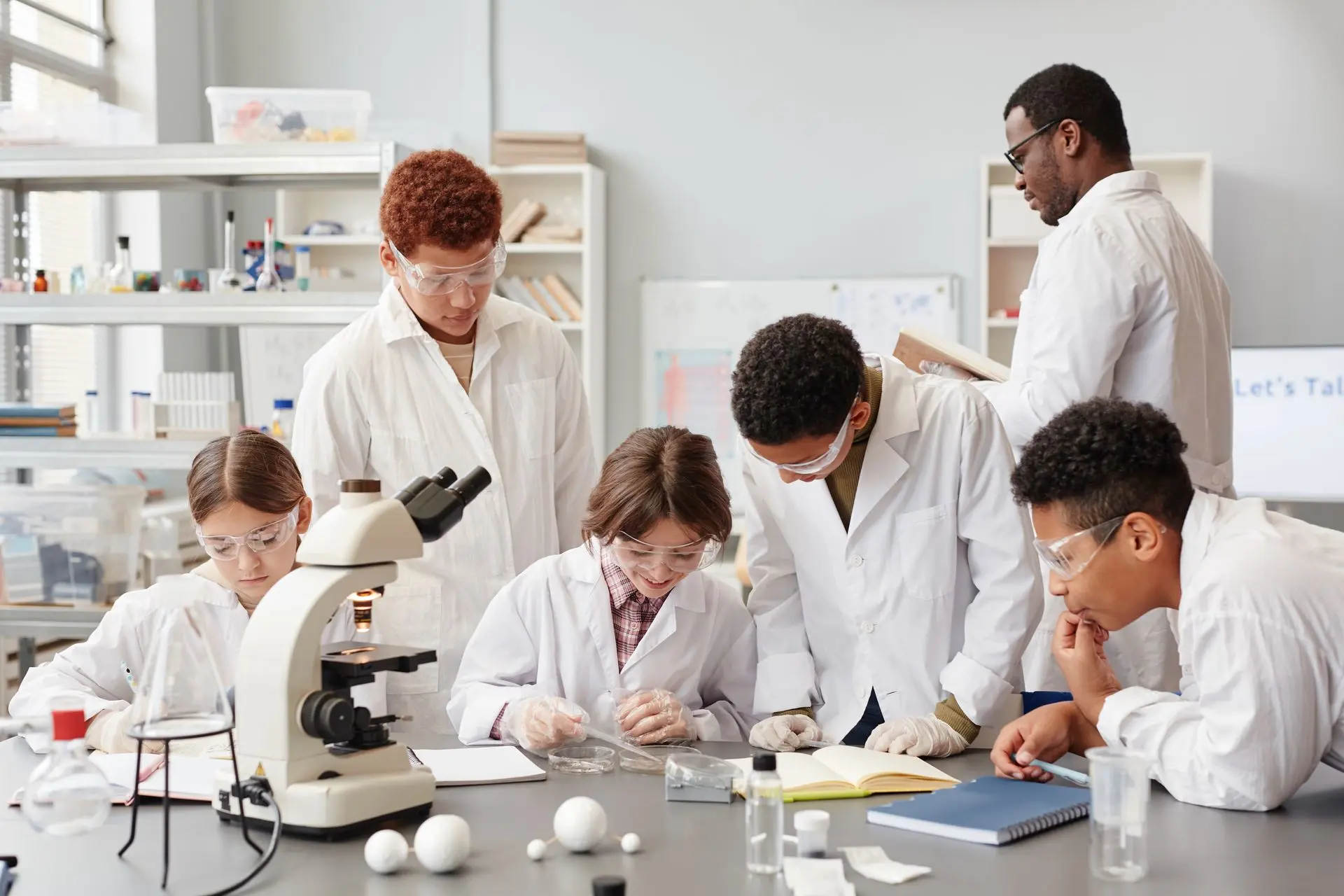
Working Groups
The Working Group 0 - "Management" stays connected with the Participants of the Action with respect to all scientific and networking activities via the National Representatives in the MC, the WG Leaders, the Contact Persons of the participating institutions, and the Action Chairs’ mailing list. Management includes soliciting new participants, interacting with industry, decisions on and allocation of funds, coordination of dissemination activities, and coordination of the networking activities.
For the Working Groups 1 - "System design and nanofabrication", 2 - "Physical processes and modelling", 3 - "Improving spectroscopic techniques", and 4 - "Preparation of a coherent textbook on optical nanospectroscopy", one WG Leader and one Vice WG Leader each will be elected by the MC. They will streamline the scientific research of the WGs, distribute appropriate calls for research proposals, and annually give progress reports on the WG results to the MC and Action Chairs for dissemination. Where appropriate, the WG Leaders may schedule individual WG meetings. Each Participant of the Action will indicate his or her affiliation with one or more of the WGs to the Action Chairs.
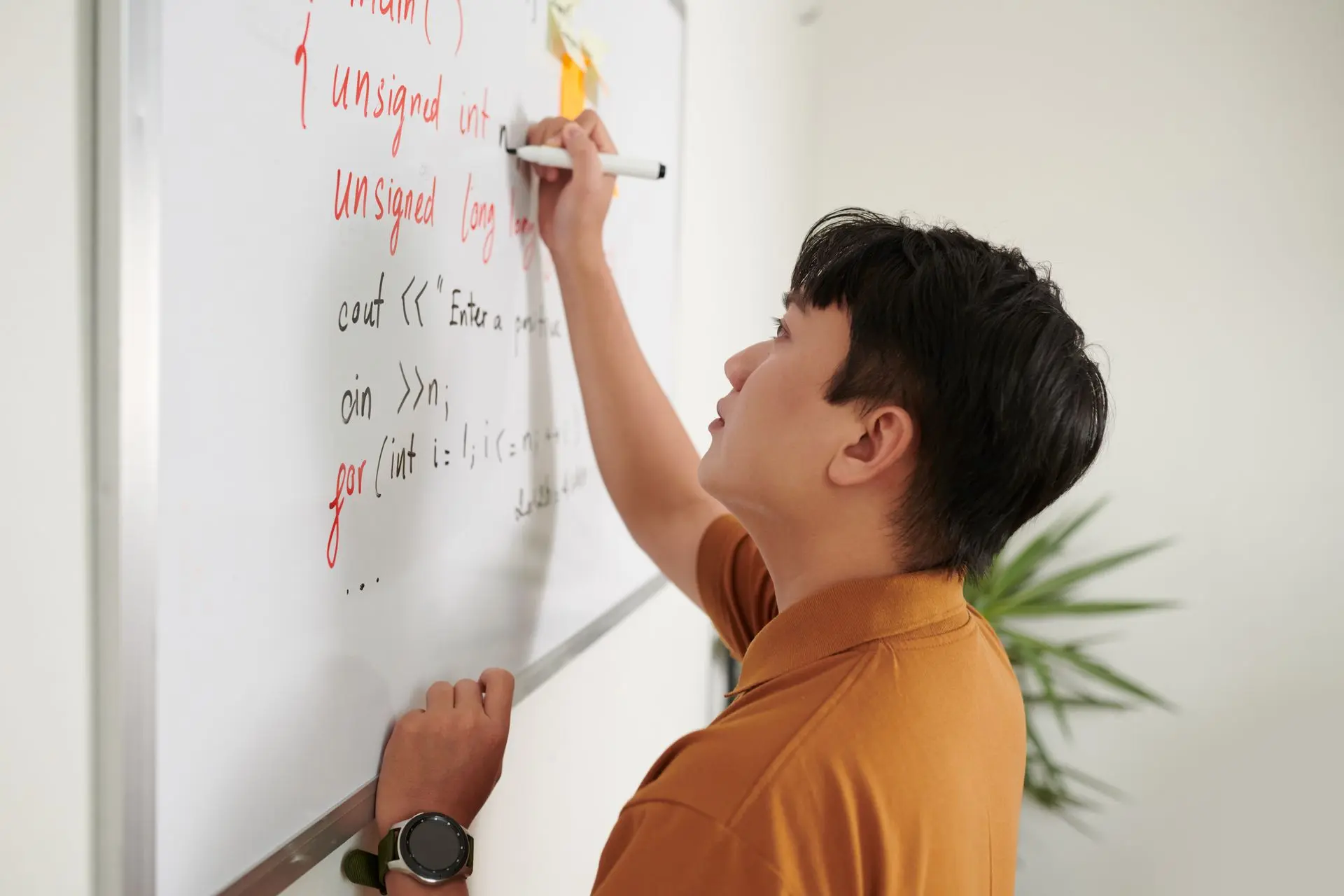
Liaison and Interaction with other Research Programmes
Interaction with FP 7 / Horizon 2020 research projects dealing with aspects of nanospectroscopy will be sought by searching the database for relevant projects and addressing the corresponding researchers as potential additional participants of COST NanoSpectroscopy or external speakers at NanoSpectroscopy workshops. COST Action MP1102 microCoR partly pursues similar objectives as the Action NanoSpectroscopy using microscopy with a specific coherent Raman microscopy approach. A joint seminar may be suggested to the MC.
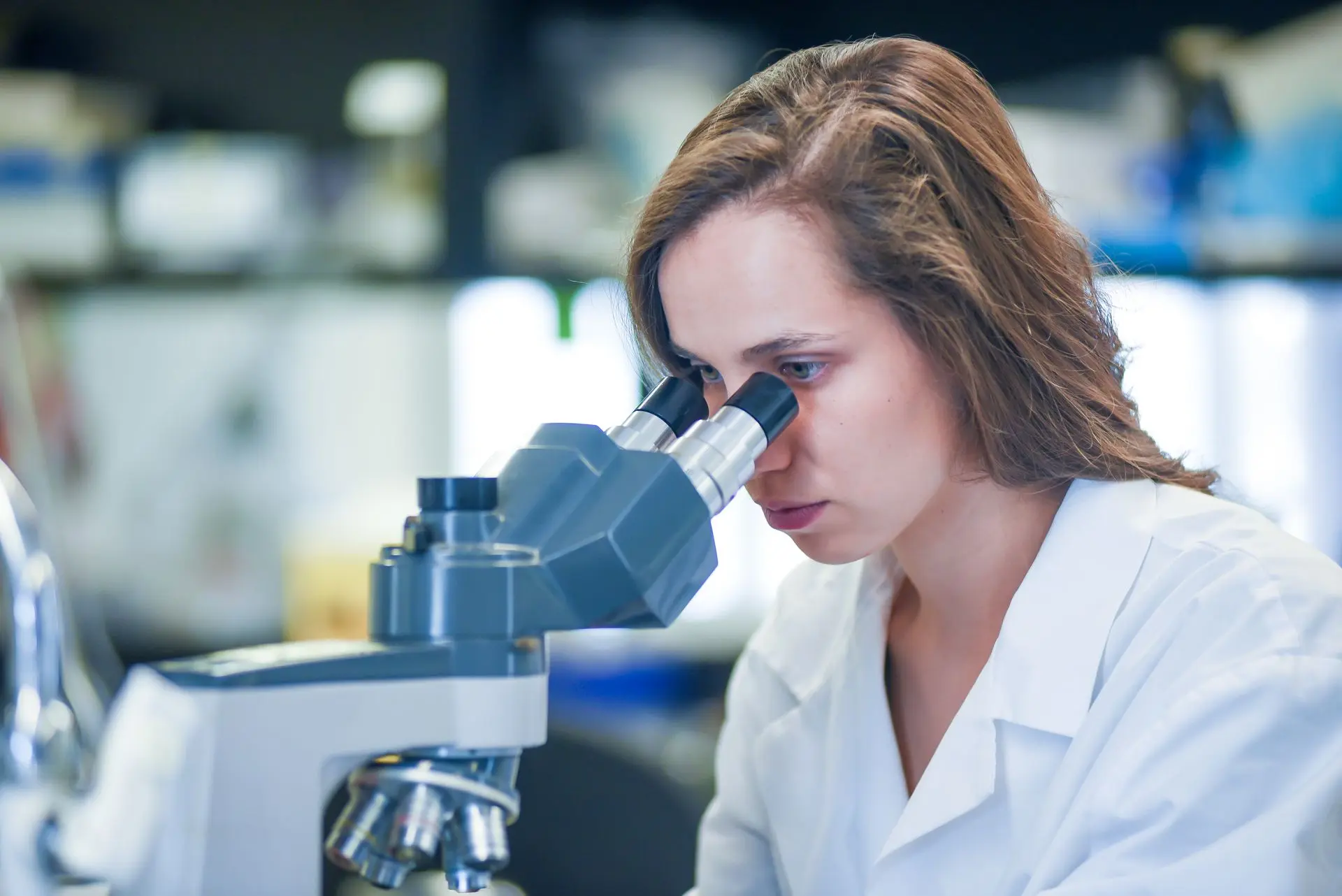
Gender Balance and Involvement of Early-Stage Researchers
This COST Action will respect an appropriate gender balance and considerable involvement of early stage researchers (ESR) in all its activities and the Management Committee (MC) will place this as a standard item on all its MC agendas. Particular care will be taken to personally address and actively recruit female scientists and ESRs. For this purpose, suggestions will be collected from the existing Participants, and the speaker lists of conferences and workshops on nanospectroscopy-related topics will be consulted. Gender balance and the involvement of ESRs will be taken into account during the planning of networking activities such as the annual NanoSpectroscopy workshop, and external speakers may be addressed to extend the scope of the COST workshop. Training courses will specifically address ESRs. In workshops organized by ESRs for ESRs, they will have full control over the contents of the activity and the additional benefit of a strong learning experience involving organizational soft skills. The distribution of funds will be monitored to ensure that ESRs benefit in particular from the networking activities and Short-Term Scientific Missions, and that no gender bias becomes evident in the distribution.
Scientific Focus
The scientific programme concentrates on nanospectroscopy in the UV/Vis/NIR spectral range, with a special focus on technique development, basic understanding of light-matter interaction at the nanoscale, and on (supramolecular) nanostructured hybrid materials. The scientific projects will be financed via individual or joint national research grants attracted by the Participants. The Action will coordinate nanospectroscopy activities under different interdisciplinary aspects:
Improving spectroscopic techniques with spatial resolution beyond the diffraction limit, with high spectral and/or temporal resolution and sensitivity down to the single-molecule level: A key factor will be the further development of real-time data processing and evaluation, requiring expert knowledge of light-matter interaction. Development of turn-key techniques will be crucial in making nanospectroscopy a daily workhorse in applied science and industry, with expected high societal impact.
Understanding and modelling basic physical and chemical processes of light-matter interaction in nanostructured systems and devices on the nanometre scale: The nanospectroscopic data will be analysed by means of physical and chemical models, and compared with theoretical/numerical models.
System design and nanotechnology includes top-down and bottom-up fabrication of organic, inorganic, and hybrid nanosystems. Advances in nanotechnology and (supra)molecular engineering are the prerequisites for investigating new thin-film and nanostructure geometries and compositions and optimizing their optical/electric properties.
Device applications: This Action will coordinate the application of the knowledge gained on e.g. electric field distributions and energy/charge flow on the nanoscale for proof-of-principle devices such as photovoltaic cells, (bio)sensors/diagnostic devices, or lasers.
Workplan
Instrumentation development: UV / vis / NIR nanospectroscopic techniques with improved (spatial, spectral, temporal) resolution and sensitivity, in-situ data processing and analysis will be a major issue of this Action. In discussion with experts on home-built setups and with industry, open issues of instrumentation development will be identified, and where possible addressed, e.g. by the development of novel near-field optical scanning probes, or turn-key techniques to address a wider group of users (biology, medicine, industrial analysis).
Materials and devices: Nanomaterials with interesting properties for the nanospectroscopic investigation of light-matter-interaction will be developed. Single- and dual-component semiconductor quantum dots and colloidal metallic nanoparticles with variable shapes will be synthesized. Single material and multilayer metallic nanostructures of various shapes, and metal-semiconductor hybrid layered structures will be fabricated using nanolithography. Nanoparticles and nanostructures will be assembled in dimer- and heteromer-configurations with small gaps for strong electromagnetic coupling using self-assembly, self-aligned fabrication strategies, and nanolithography. Organic single- and multi-component systems (nano- or single crystals, thin-films) will be prepared by (supra)molecular engineering. Carbon allotropes will be integrated using commercially available or chemical vapour deposition of carbon nanotubes, fullerenes, and/or graphene. Hybrid organic-inorganic systems will be prepared e.g. by coupling molecular, e.g. protein, systems to metallic optical antennas, by implementing metallic or semiconducting nanoparticles in organic matrices, or by local near-field photopolymerization using the electric near-field of metallic particles. The findings on both energy flow and material optimization will be implemented for the proof-of-principle demonstration of enhanced device properties for applications such as plasmonic photovoltaics and (bio)sensing/diagnostics.
Nanospectroscopic investigation and modelling: The materials will be investigated using a variety of nanospectroscopic techniques specifically adapted for their high temporal, spatial, and spectral resolution analysis. A non-exclusive overview over such methods is listed in section Methods and Means.
Nanospectroscopic investigation and data analysis will be undertaken and supplemented by theoretical modelling. The scientific aim is to advance knowledge on topics in the field of light-matter-interaction and energy/charge flow on the nanoscale such as:
Characterization of the absorption and emission properties of novel and hybrid nanomaterials
Time-resolved detection of energy and charge transfer processes at the nanoscale
Understanding and improving light harvesting processes and efficiencies
Structure-property-relationships in conjugated organic materials and carbon allotropes
DNA imaging, dye-DNA interactions
Exciton control and design rules for better lasing materials
Nanostructured multi-chromophore materials: unprecedented effects by cooperative interaction
Understanding of interactions of carbon nanotubes with polymeric environments
Far field / near-field optical properties of single/coupled metallic nanoparticles
Molecular plasmonics : interaction of metallic nanoparticles with passive/active molecules
Excitation/emission enhancement mechanisms in metal/surface enhanced fluorescence
Optimization of surface-enhanced Raman spectroscopy and (localized) plasmonic sensors.
The human and technical means needed to achieve the objectives in C are provided by the Participants of the COST Action NanoSpectroscopy. Sufficiently broad expertise is present within the Action. However this Action is expected to be interesting for a much larger number of European researchers. Additional Experts can easily be included in the flexible work plan and will strengthen the collaborative effort in pursuing the same objectives on a bigger scope. The workload of organizing workshops and training days will be shared between the participating countries and Working Group Leaders. Technical means for achieving the objectives are mostly clean room and nanofabrication facilities as well as optical setups and laser benches in optical spectroscopy laboratories. All relevant technology as detailed in D.2 is present in the Action. As one advantage of the Action, the equipment will be made available across the groups, leading to synergistic effects. Groups interested in joining this COST Action will contribute their own technical means. For the publication of the nanospectroscopy textbook, renowned publishing houses were already addressed and have expressed interest.
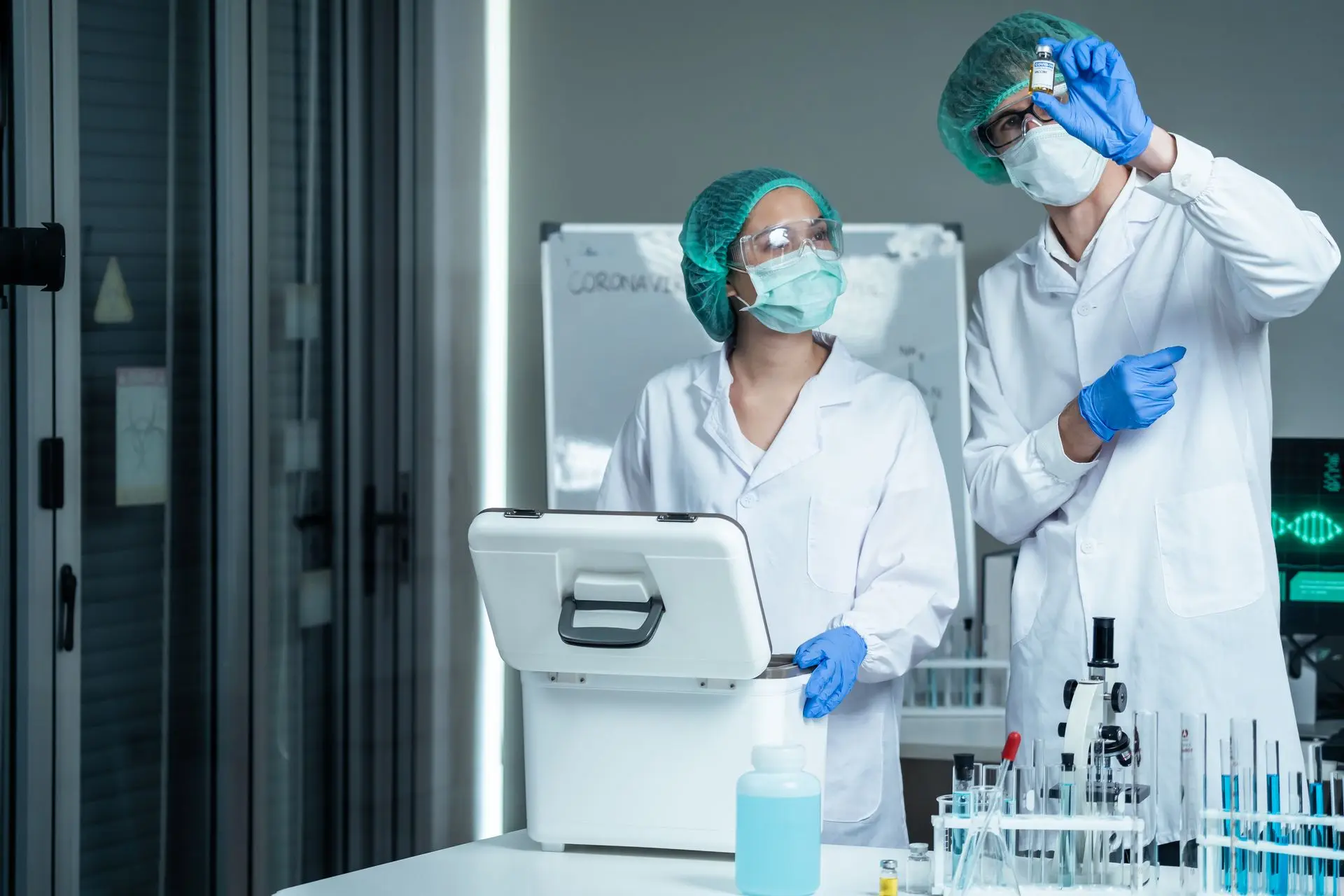
Scientific Work Plan Methods and Means
To implement the scientific work plan, one needs to distinguish between methods and means for designing the nano-systems of interest, methods and means for their nanospectroscopic investigation and modelling, and methods and means of improving the spectroscopic techniques.
Nanosystems design within this Action will include, but not necessarily be restricted to, molecular engineering by targeted synthesis of pi-conjugated polymers and oligomers, cyanine dyes, organic single crystals, supramolecular nanostructured multi-chromophore materials, organic nanoparticles, channel-forming host-guest compounds, organic bulk heterojunction photovoltaic blends, carbon nanotubes in polymeric environments, ferroelectric insulators, fluorescent proteins, biological materials, self-organization of self-assembled monolayers, chemical vapour deposition and molecular beam epitactical growth of II-VI and III-V thin films, colloidal nanoparticles, semiconducting and core-shell nanocrystals, size-selected nanoclusters, top-down nanofabrication of organic/inorganic insulating, semiconducting, metallic, and hybrid nanostructures by electron beam lithography, focused ion beam milling, nanosphere lithography, and nanoimprint lithography. The constituents will be combined to form hybrid materials, and individual elements will be positioned relative to each other by defined placement, lithographic overlay, and self-aligned attachment. The nano-elements may be integrated using optical lithography, metallic contact thin-film deposition, or dielectric embedding, to measure device characteristics. As a means to obtain these structures, clean room facilities will be required. The necessary equipment includes spin-coaters, Langmuir troughs, glove boxes, thin-film evaporators, scanning electron microscopes, dual beam / focused ion beam machines, chemical vapour deposition or molecular beam epitaxy machines, colloidal synthesis flasks, cluster beam deposition, and imprint presses.
Nanospectroscopic techniques that will be applied and further evolved will be based on light sources operating in the ultraviolet, visible, and near-infrared frequency range. Methods available through the applicants include extinction/transmission spectroscopy, total internal reflection spectroscopy, dark field scattering spectroscopy, confocal laser spectroscopy, micro Raman spectroscopy, surface-enhanced and tip-enhanced Raman spectroscopy, pump-probe laser spectroscopy, higher harmonics generation, Fourier-transform infrared spectroscopy, super-resolution and single molecule fluorescence spectroscopy, fluorescence and nanoparticle resonance energy transfer, fluorescence excitation spectroscopy, metal/surface enhanced fluorescence, time-resolved ultrafast / pump-probe spectroscopy, time-correlated single-photon counting, femto-second fluorescence up-conversion, pulse-gated detection, electro-absorption spectroscopy, high spectral resolution nonlinear spectroscopy in the frequency and time domains (spectral hole-burning, optical coherent transients, fluorescence line narrowing), optical-magnetic double resonance spectroscopy, low temperature confocal single-molecule spectroscopy, luminescence emission of plasmonic nanostructures, fluorescence polarization microscopy, and more.
For the theoretical modelling of electromagnetic field distributions as well as charge and energy transfer on the nanoscale, commercial programs and personal coding will be employed, using e.g. density functional theory (DFT), multiple multipole programs (MMP), finite-difference time-domain methods (FDTD), finite element methods (FEM), or finite integration techniques (FIT).
To improve nanospectroscopic instrumentation, initially a list of open issues will be drafted and disseminated. This will include questions of reproducibility, high-resolution large-area imaging, integration times, robustness, data analysis, light sources, etc. As one aspect of instrument development, novel tip-enhanced near-field scanning probes will be developed and evaluated in a tip-enhanced Raman spectroscopy setup. Further topics may be addressed depending on the scope of the Participants and interested companies.
Working Groups
The following Working Groups (WGs) will be established to address the differentaspects of the work plan:
WG 0 - "Management": The WG "Management" stays connected with the Participants of the Action with respect to all scientific and networking activities. Management includes soliciting new participants, communication with industry, decisions on and allocation of funds, coordination of dissemination activities, and coordination of the networking activities.
WG 1 – "System design and nanofabrication": The Action will focus on molecular engineering and on hybrid combinations of inorganic/organic, semiconducting/metallic or metallic/metallic nanomaterials. Top-down and bottom-up fabrication of novel well-defined nano-systems and the synthesis of new promising moleIV and II-VI nanocrystals, III-V compounds, specifically designed organic dyes, oligomers and polymers, carbon allotropes, nanoclusters, Au/Ag colloidal particles, and tailored layered, core-shell, or bimetallic nanostructures in different shapes. The different constituents and combinations of the constituents and devices will be provided to WG 2 for investigation and will be further optimized based on the results.
WG 2 – "Physical processes and modelling": To achieve a more in-depth understanding of the transfer of energy and charges in hybrid nano-systems, emission and absorption properties, hybridized states arising from coupling as well as local field effects, such phenomena will be studied experimentally in suitable systems and modelled theoretically (by DFT, MMP, FDTD, REM, FIT, etc.). Interactions will be investigated down to the single hybrid nano-object level. A special focus will be maintained on energy transfer in view of improved device properties for light management (LEDs, photovoltaics, spasers). Materials with promising energy transfer properties will be identified and optimized with respect to the main characteristic parameters.
WG 3 – "Improving spectroscopic techniques": To improve spectroscopic techniques, issues of reliability, reproducibility, large area applications, and data analysis will be addressed. As one example, innovative nanoprobes for tip-enhanced Raman spectroscopy will be fabricated and evaluated. Vendors of spectrographs, microscopes, and optical components will be invited to collaborate.
WG 4 – "Preparation of a coherent textbook on optical nanospectroscopy": A coherent table of contents for a nanospectroscopy textbook aimed at ESRs will be devised by this WG and used for approaching renowned publishers. Individual chapters will be assigned to Experts within the Action. The textbook will be published as one objective of this Action.